It seems helpful to illustrate cosmological scale apparent aging as stars depart main sequence:
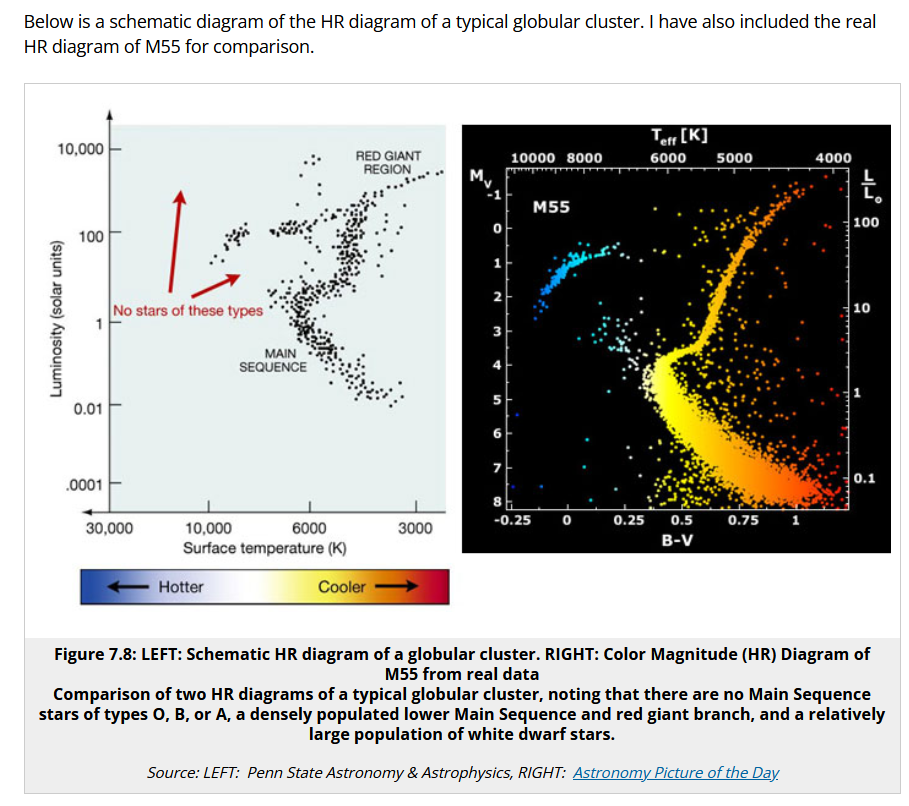
An idealised, Hertzsprung-Russell chart for Hydrogen-rich balls prone to become fusion furnaces is:
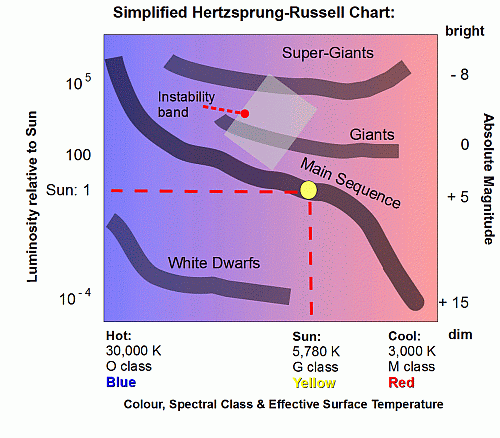
Here is a comparative plot (for open clusters), constructing a “clock” by projected pattern as a cluster ages, in effect seeing what is left as a candle burns down:

This can be taken as illustrative of how our cosmos shows entropy-associated aging on the grand scale.
Further illustrative, here is a NASA-derived cosmological timeline model, integrated with fine tuning:

Speaking of fine tuning, Barnes et al summarise:

All of this ties to core thermodynamics:

Food for thought. END